John Whitehead, PhD
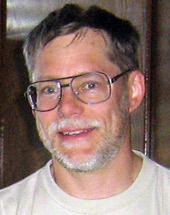
John Whitehead, PhD, is an aerospace engineer who currently works to advise a diplomatic team dedicated to reducing the proliferation of destructive technologies around the world. In recent years he also has applied his expertise in orbital dynamics and space propulsion to the challenge of maintaining satellite safety as earth orbit becomes more crowded.
The freedom to pursue his own interests as a Hertz Fellow helped to start him on a varied career somewhat outside the mainstream.
A passion to build tiny 100-mile-per-gallon cars circa 1980, combined with the stimulus of graduate coursework in dynamics and controls, led John to an improved understanding of steering stability. He published new equations and diagrams that explain a contributing physical reason why it is easy for drivers of wandering vehicles to “overcorrect” from a steering error and run off the road on the opposite side. Such single-vehicle crashes appear regularly in news articles, with little in the way of explanation. In the absence of an easy fix, the natural characteristic inherent to cars and trucks has not been widely discussed.
Earlier while an undergraduate in biology at Caltech, learning about the great discoveries of the past impressed upon John the value of seeking simple explanations for seemingly complex phenomena. After a switch to engineering, graduate school at UC Davis provided exposure to the notion that mathematical modeling should include as much detail as possible, in order to be most realistic. In all subsequent work, he has sought a balance between the two approaches.
While transitioning from his BS in biology to another in mechanical engineering, he co-founded a student team that later sent his plant physiology experiment to orbit on an early space shuttle trip in 1983. After specializing in dynamic systems and control theory to finish his university years, he switched topics again upon receiving his PhD. During two decades at Lawrence Livermore National Lab (LLNL), he built and tested high performance rocket propulsion on a scale of kilograms instead of tons, a challenge which amounts to miniaturizing satellite launch vehicles. In 1994, his LLNL team launched the world’s smallest pump-fed rocket from Vandenberg Air Force Base.
In subsequent years he realized that his effort was on a path to enable NASA’s long sought but elusive dream to analyze Mars geology samples in labs on Earth. As explained in his publications, the latter mission needs a launch vehicle the size of a person that can quickly reach a very high velocity to depart from a planet, far more difficult than lunar return. Circa 2005, NASA funded two years of his work at LLNL. While the National Academy of Sciences has since designated Mars Sample Return as the highest priority for planetary science, there is still no sustainable research community focused on this particular rocket challenge.
Starting in the late 1990’s, some of John’s laboratory efforts were dedicated to propulsion for smaller satellites using reduced-hazard propellants. Connecting to the small satellite community, he helped to inspire others’ efforts toward maneuvering propulsion on cubesats, and for tiny rockets to launch cubesats. Both remain topics for research and development, while being somewhat elusive goals, to this day.
Also in the late 1990’s, he turned some of his attention to big challenges in space transportation. One of his papers explained why the goal of reusable single state to orbit (SSTO) vehicles remains a holy grail. Another showed how to separate physics from economics in launch vehicle cost analysis, simultaneously explaining why the “big dumb booster” idea never came to fruition. The latter concept is that launching to orbit might be more affordable if very large moderate performing rockets can be made of cheap materials, even though the payload fraction would be very small.
In a 2007 paper, Dr. Whitehead explained the fundamental limits on the speed of aircraft powered by combustion using atmospheric oxygen, and particularly why we should not expect airplanes to ever fly to orbit. The air capture area has to increase as speed squared, exceeding vehicle drag area above roughly one quarter of orbital speed. Given realistic drag coefficients, the air inlet would have to be larger than the whole vehicle. In the field of hypersonics, this fundamental limit to flight speeds has largely been overlooked during fifty years of research in supersonic combustion and high-temperature materials.