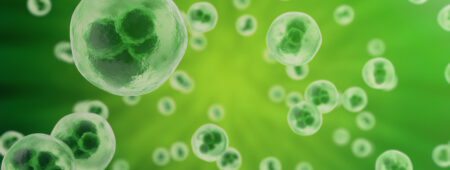
Manipulating Proteins to Create Cellular Ultrasound
Some scientists are driven by a desire to get to the bottom of things. Biochemical engineer and Hertz Fellow Mikhail Shapiro wants to get to the middle.
Inspired by a journey inside the body to remove a blood clot from the brain of a dying scientist in the classic sci-fi movie Fantastic Voyage and the writings of futurist Ray Kurzweil, Shapiro is engineering cells to produce air-filled proteins that act as biological sensors for imaging and controlling cells inside the body.
These air-filled proteins, called gas vesicles, can get to hard-to-reach places—neurons in the middle of the brain, microbes in the middle of the gut, or tumor cells inside of an organ—and enhance ultrasound for imaging inside of cells.
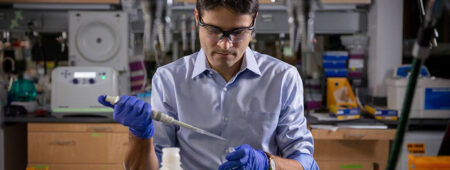
For this work, Shapiro has been recognized by Science News as one of 10 scientists selected for pushing the boundaries of scientific inquiry to solve some of science’s biggest challenges.
“Most of the amazing tools people have built for imaging and controlling cells don’t work well when they’re in the middle of the body, because light can’t penetrate there,” said Shapiro, professor of chemical engineering at the California Institute of Technology. “It's important to be able to see where cells are inside the body and if they arrived at their destination. Are they carrying out the function that we want them to perform?”
The uses for gas vesicles for advancing science are numerous. Because these tiny air-filled bubbles have the right physical properties to be picked up by sound, someday they could be a vehicle for driving therapeutics deep into tissues to diagnose or treat disease using ultrasound.
Motivated by the use of green fluorescent proteins in jellyfish to learn about gene expression and the inner workings of cells—work that garnered three scientists the 2008 Nobel Prize—Shapiro hopes to do with gas vesicles for ultrasound what green fluorescent proteins did for optical imaging.
Unlike green fluorescent proteins used in microscopy, ultrasound can easily image centimeters deep into tissues in real time, providing a wide-angle view, only it is missing a paired biomolecule to show what cells are doing. This is where gas vesicles come in.
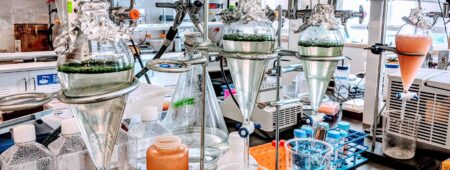
An Unexplored Path
Shapiro began his years-long focus on improving how to see inside the body as an undergraduate at Brown University, where he encountered devices that interact with neurons to beam out what is happening in the brain.
Then, during a postdoctoral fellowship at University of California, Berkeley, he read a paper that referred to an interesting biological phenomenon for maintaining buoyancy—gas vesicles discovered inside blue-green algae floating in lakes around the turn of the 20th century.
Hypothesizing about the physics of gas vesicles, which until this point had gone unexploited, Shapiro ordered blue-green algae from a lab in Scotland. Not having a growth chamber to cultivate in, he simply placed the algae inside a flask under a fluorescent light above his workbench.
To his surprise, the algae grew, and he extracted the air-filled proteins from its cells. Using an abandoned piece of ultrasound equipment hauled up from the lab’s basement, Shapiro discovered that the gas vesicles produced a signal and were detectable with ultrasound, confirming his hypothesis.
“If I hadn’t read those few sentences about gas vesicles, we wouldn’t be here today saying that it is possible to do biomolecular ultrasound,” Shapiro said. He has since engineered the program for inserting the gene from gas vesicles into different cells and hopes to see acoustic proteins used someday to develop cell-based immunotherapies and diagnostics for brain disease and other illnesses.
“In 10 years or more, I'll consider ourselves a success if every biology lab around the world has an ultrasound system next to their optical microscope, and doctors are administering cell-based therapies to people using ultrasound, sending commands to release their therapy inside the body,” he said.
Because it’s so new, his work is unique among his colleagues, but a worldwide network of collaborators, including former graduates from his lab, is developing.
His students, he says, are what keep him motivated. He cites his own graduate school days and the freedom of the Hertz Fellowship as pivotal to his work today. The mindset of the fellowship pushed him to pursue his ideas to their furthest conclusion.
Now, as an interviewer for the fellowship, he sees the benefits of learning the science fellowship candidates are interested in, from artificial intelligence to genome editing.
“It’s a way to get a pulse of what these smart young people see as the future,” he said.