Scientists Invent New Method for Improving DNA Nanotechnology Designs
In the budding field of nanotechnology, one of the most versatile building materials explored in recent years is DNA – the same molecule that carries our genetic code. DNA nanostructures are built from custom-made DNA strands, which fold and assemble together into almost any shape imaginable. Scientists hope to make structures that can do everything from molding futuristic materials to carrying lifesaving medicine directly to cells. The challenge in building with DNA is that the strands don’t always stay connected – more often than not, the structures fall apart.
Hertz Fellows Michael Baym and Cameron Myhrvold think they have a way of preventing just that. Together with their colleagues at Harvard University, Baym and Myhrvold have invented a method of finding the weak points in DNA nanostructures. Called BEARS, for “Barcode Extension for Analysis and Reconstruction of Structures,” the method was published March 13 in Nature Communications.
The BEARS method takes advantage of the fact that each of the hundreds of tiny strands that makes up these structures has a unique sequence. By finding which sequences are incorporated into the structure, as well as which sequences are not, the method quickly identifies exactly where on a structure the design might need to be improved. “It’s a way of using the sequencer as a microscope,” says Baym.
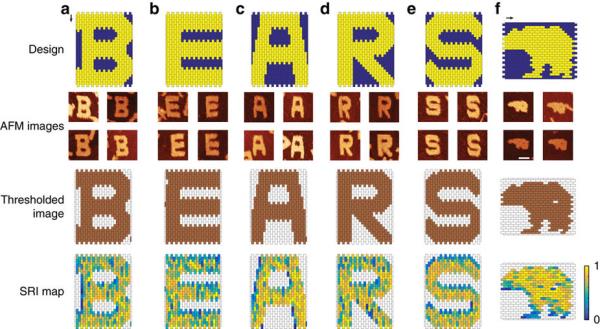
The collaboration was born of a fortuitous conversation one Friday in the fall of 2013. “Cameron and I were at a happy hour, having a beer,” recalled Baym, who was then a postdoc at Harvard. “I don’t know how the topic came up,” he says, but somehow the conversation turned to Myhrvold’s frustration with DNA nanostructures.
“Working with nucleic acids [like DNA and RNA] is a really elegant way to build things,” says Myhrvold, who performed the work as part of his PhD from Harvard University. By customizing the long strands’ sequences, scientists can find exactly which strands tend to stick together and where. Just mix the different DNA strands in hot water, then cool slowly as the strands find the places where they best stick together.
In theory, says Myhrvold, “you’re limited only by what you can design.” The resulting assemblies range from intricately folded “DNA origami” to structures built of hundreds of tiny “bricks,” with each brick made of a single DNA strand. Some structures are already being tested for ferrying precise drug doses to cells.
But that September as he met Baym at happy hour, Myhrvold felt that there was something holding him back: how to quickly and easily check whether structures actually assembled into their designed shape.
“I’d been frustrated by the way we characterize DNA structures,” says Myhrvold. “It hasn’t changed in a long time.” Advanced microscopes can zoom in on structures less than one percent the width of a human hair and see if they are missing large chunks or arranged incorrectly. But these microscopes are often very difficult or expensive to use to their full potential – and they can only look at one structure at a time. Myhrvold wondered whether, instead of using microscopes, he could use DNA sequencing to simply “read” the sequences of DNA that did – or didn’t – make it into the completed structure.
Baym studies antibiotic resistance, so he spent a lot of time sequencing the DNA of different bacteria to track how genes for resistance spread. As the two talked, they realized they could work together to make a breakthrough. “We decided it might not actually be that hard,” said Baym. “The sequencer doesn’t care what the DNA comes from,” so it would be just like reading the bacterial DNA that Baym reads all the time.
The method they came up with first separates DNA by size, then reads it. After the DNA strands have assembled – or not assembled – into structures, they are pushed through a gel with an electric current. Small fragments and single strands move easily through the gel, grouping up at one end, while nearly complete structures lag behind at the other end. The structures are then cut out of the gel, labeled with short sequences of DNA (the “barcodes” that give BEARS its name), and given to the sequencer for reading. The less often a strand showed up with the completed structures, the more likely it was to be a weak point in the overall structure.
To test the method on an entirely new structure, the two designed a flat structure of DNA bricks that looks like a pixelated picture of a bear. “Michael and I are both bear enthusiasts,” explained Myhrvold. “I like that they’re simultaneously ferocious and cute.” Between experiments, the two would watch Bear Cam, a livestream of bears fishing for salmon in Katmai National Park.
Their sequencing test showed that one of the bricks near the bear’s rump, along with a few near its front leg, were prone to falling off. Sure enough, when they looked through the microscope, many of the microscopic bears had a hole in their rump or were missing their front legs. Those DNA strands were just not sticking together as well as others.
The applications range far beyond microscopic bears. Myhrvold and Baym also used it to see which strands were falling out from the inside of a square tube they constructed, showing that the “microscope” of sequencing can peer inside the structures. And the method is so cheap and easy to use with existing technology that other scientists were asking to use it on their own structures even before the paper was published.
Myhrvold says the experience changed how nanotechnologists like him think about DNA sequencing. “A lot of people in the nanotechnology world aren’t really familiar with sequencing,” says Myhrvold. “It seemed like this new, weird crazy idea.”
But it worked – and the work affected Myhrvold’s career path. Now a postdoc at the Broad Institute, he studies how viruses evolve – and whether we can guide that evolution to prevent outbreaks. To track how the virus’ genes change, he says, “sequencing is hugely important.” The BEARS project, he says, “set me up nicely to dip my toes into the whole sequencing thing.”
For Baym, too, BEARS has inspired future work. He began his career as a theoretical biologist before making the slow transition to working in the lab, thanks in part to the freedom granted by his Hertz fellowship. “It turns out experiments are really fun,” he says. Now, Baym is preparing to start his own lab at Harvard Medical School, where he will study antibacterial resistance. He says using sequencing as a sort of microscope has inspired him to think of the technique in different ways. “What this really drove in for me was the versatility of DNA sequencing as a probing technology beyond just genomes,” he says. “Any information encoded in DNA or RNA is out there for the taking. It’s very empowering.”